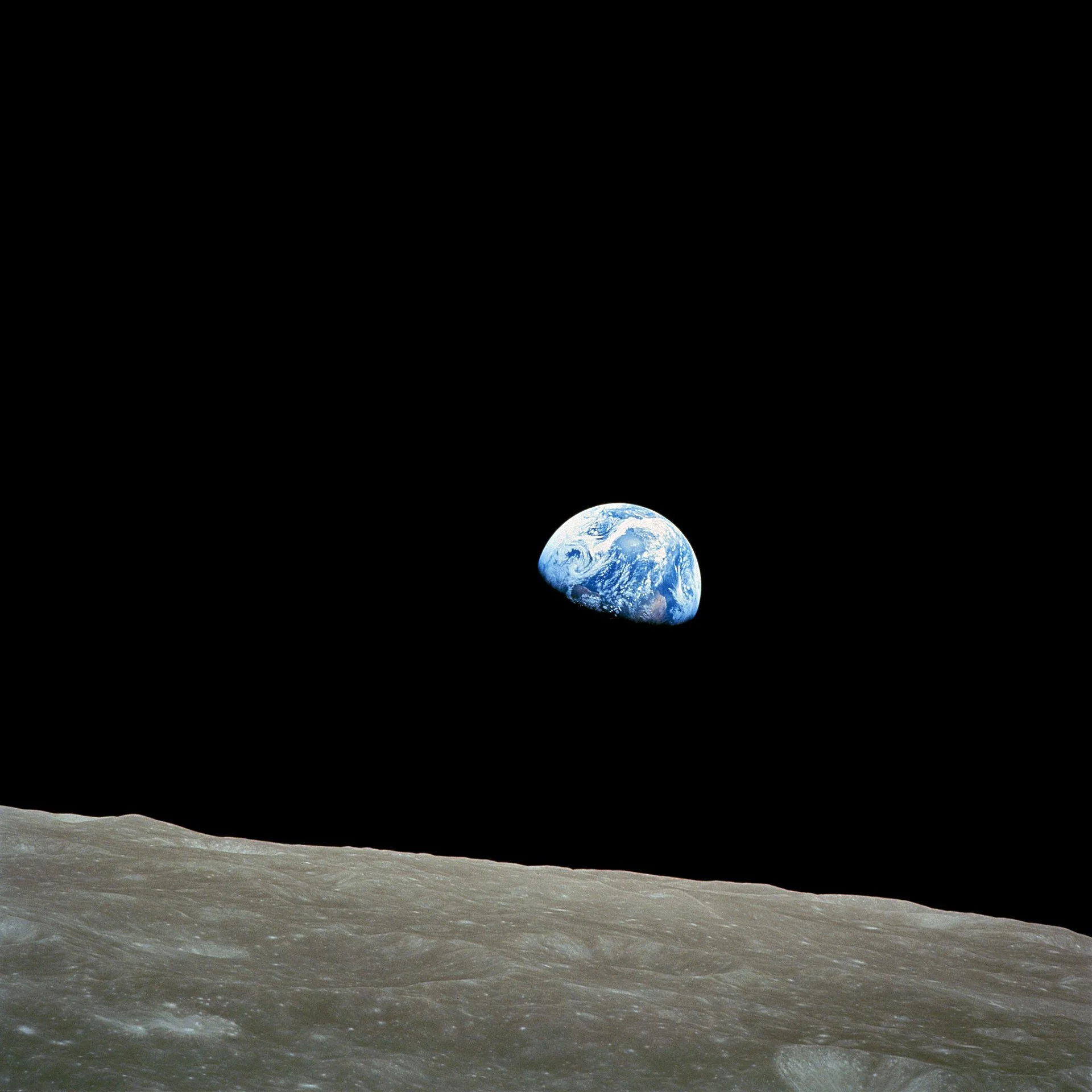
Using Space
Resources
for Benefits
on Earth
Earth rise 1968 Apollo 8 by William Anders
Developing advanced concepts for space utilization and benefits on Earth .
We are currently preparing conceptual designs, simulations and focused tests and demonstrations for an all reusable lunar lander prospector, artificial gravity space habitats and an all reusable spaceplane. We are using Ansys/AGI System Tool Kit (STK) for in-space simulations and SolidWorks for computer aided design. When the simulations and designs can claim feasibility, we will publish results and discuss system development with government agencies and industry. The artificial gravity space station conceptual design has matured nicely and we are now sharing a whitepaper with the government and industry. We have responded to the Office of Science and Technology Policy (OSTP) requests for information for Cislunar Technology Priorities and Sustainability of Microgravity R&D. Summaries are posted at the bottom of this website. Full responses are available upon request.
Contact Us
martin@mclaerospace.com
(817) 239-0198
Space Resources
The following is a list of space resources, their unique features and some potential applications. Questions, comments and suggested other resources are always welcome.
Space Resource, Unique Features and Potential Applications
Solar Power: Space solar power has constant flux without diurnal or atmospheric attenuation. Very large solar farms can be constructed in space for unlimited solar power collection and concentration.
Micro-Gravity: Micro-gravity in space enables the construction of very large low mass structures. Many other activities are enabled by micro-gravity including growing large crystals, unique micro-biology and others yet to be imagined.
Artificial Gravity: Rotating platforms with artificial gravity. Spin rate adjusted for Earth, Mars and Moon gravity or anywhere in between. Provides control runs and analysis instruments that require gravity for microgravity research near real time rather than waiting for sample return to Earth. Provides long duration crew health and comfort. Can recondition crews after long low gravity missions for safe Earth return analogous to diver decompression chambers.
Hard Vacuum: Space vacuum is much lower than the most sophisticated laboratory and industrial vacuum chambers on Earth and is free. Hard space vacuum enables very high purity micro-chip and material processing and has unlimited scale up potential. Chemical vapor deposition could become a major in-space manufacturing process for very large high purity fabrication.
Lunar Resources: Our moon is close by with a low gravity well for access to bulk materials, volatiles and rare Earth elements. Our moon has 100s of millions of accessible asteroid and comet impact craters with materials representing the entire solar system. Our moon has been dusted by solar winds for billons of years and unique materials with atomic chemistry reactions that occur only in the sun’s atmosphere, and are accessible and undisturbed in the first meter of the lunar surface.
Asteroid Resources: There are many more asteroids nearby or that transit cislunar space than previously understood. These asteroids are potentially accessible and certainly much easier to reach than those in the asteroid belt beyond Mars. These more accessible asteroids have bulk materials in a very low gravity well and likely have valuable rare Earth elements.
Expended Spacecraft: Since the 1960s there are more and more expended launch vehicle upper stages and spacecraft in Earth orbit and cis-lunar space. Aerospace grade materials can be harvested from these vehicles and some of their components might be repurposed.
Solar Wind: The solar wind could be useful as a low force shaping and steering force for micro-gravity manufacturing. Electronic charge could accumulate on items subjected to the solar wind. This could be both a problem and an opportunity.
Solar Radiation: Our sun’s solar radiation is a full electromagnetic spectrum. Portions of that spectrum can be used for specific purposes such as UV curing polymers.
Gravity Gradients: Earth and lunar gravity gradients will continue to be useful for spacecraft stabilization for Earth and in the future lunar nadir alignment.
Magnetic Fields: Space magnetic fields have varying intensity by location. It may be possible to use space magnetic fields in the generation of electricity.
Dark Sky Heat Sink: The deep space dark sky is a 6 degrees Kelvin and will continue to be a valuable heat sink and the cold end of heat engines.
Star Field Reference System: The fixed star field will continue to be a valuable resource for navigation and control. It may also be used as nature’s theodolite for large scale space manufacturing and construction.
Galactic Cosmic Rays: Galactic cosmic rays cause biological mutations that may be useful for bio-medical research. They are also ubiquitous and isotropic which might be useful as a background.
Waste Disposal: Dangerous waste products can be placed in disposal trajectories never re-contact cislunar space or the Earth.
Nuclear Safety: Nuclear fission and fusion reactors can be operated in space with no danger to the Earth. These reactors could be beamed energy power platforms providing pollution free power to systems in cislunar space, on the lunar surface and possibly back on Earth.
Unique Isotopes: Unique and or dangerous isotopes may be recoverable on the lunar surface or in cislunar space and utilized in applications too dangerous for on Earth.
Gravity Wells: Earth, lunar and other solar system body gravity wells will continue to be useful for gravity assist trajectories and the Oberth effect.
Atmospheres: Earth, Mars, Venus and other solar system body atmospheres will continue to be useful for spacecraft aero-braking and aero-capture. They also have useful gasses for many applications.
Lunar Far Side: Due to our moon’s tidal lock, the lunar far side is a unique environment with a interference free radio frequency environment for radio astronomy and other applications. The lunar far side is primarily an Earth free environment which may be useful as a base or foundation for comparison.
LaGrange Points: The Earth-Moon and Sun-Earth LaGrange points will continue to be useful for stable minimal station keeping orbits. EML-4 and 5 may be particularly useful since the solid angle for the sun, Earth and moon are nearly the same there which could facilitate sun, Earth & lunar heat shades with a large field of regard of the dark sky heat sink. This could facilitate low boiloff low power demand cryogenic propellant depots.
Lunar Lander/Prospector Concept Study
Beamed energy for power and propulsion has the potential to enable a high performance all reusable single stage lunar lander/prospector. Off loading the energy source, combustion devices and oxidizer yields specific impulse in the 750-1000 second range for hydrogen propellant heated in a beamed energy thermal rocket engine to 2,000 to 3,000 Kelvin. Engine thrust to mass ratios are similar to chemical rocket engines and more than adequate for the lunar gravity well. The Design Reference Mission (DRM-01) shown below is very stressful with very high power requirements. But the concept does close with 1,000 kilograms of down and up mass payload. The next step is to use a simpler DRM with minimum useful payload. Comments and suggestions especially on the minimum useful payload and hop capability are desired.
Many other beamed energy applications in cislunar space and the lunar surface are possible and can share the same energy platform.
Artificial Gravity Space Station
Spinning space stations for artificial gravity have been suggested since the 1920s by Konstantin Tsiolkovsky, Wernher Von Braun, Arthur C. Clarke, Gerry O'Neil and others . A spinning space station was featured in the 1968 film 2001 A Space Odyssey. All of those were very large and complex and would likely not be practicable to launch from Earth. They will likely have to wait until the availability of in-space manufacturing with lunar or asteroid construction materials.
Our concept study is developing a simple dumbbell configuration that can be launched in just three Falcon 9 launches. Our concept includes isolation bearings for non-spinning laboratories at the station centerline. This enables precision microgravity scientific and commercial research. Researchers can spend very long durations on station without loss of condition for return to Earth.
The station can be spun at different rates for Mars or Lunar gravity. The habitats and laboratories can be reconfigured for the specific mission needs.
We are using AGI STK software to evaluate low Earth orbits, high Earth orbits and cislunar locations such as the Earth Moon LaGrange points. The user experience is also being evaluated by adding various spin rates, pointing angles and perspectives in the various compartments in the station. A subscale test and demonstration of the spin isolation bearing is in preparation.
Screen capture of sunrise from the Space Station configuration SS-02a in a 116 degree inclination orbit, at 3,385 kilometer altitude with the spin access pointing at the center of the Earth. (ANSYS/AGI STK simulation)
This is a video capture of a physics based simulation using ANSYS/AGI STK simulation software. The video shows the Earth moving along with the space station orbit and the spin of the station to create artificial gravity as if on Earth. The point of view is as if you were in the right hand habitat. For this case the station is in a polar orbit at 500 kilometers altitude and the station is spinning with the spin axis pointed at the center of the Earth at 4.7 rpm. You can see Cape Canaveral and Central Florida as the station's orbit progresses southward. The ground is spinning from the point of view of a person in the right hand habitat. Please submit your questions, comments or suggestions.
This is the same orbit as the previous video except looking up toward space.
We are collaborating with Dr. Tammy Chang, Professor of Surgery at the University of California at San Francisco where her laboratory conducts liver tissue engineering in simulated microgravity. She has shown that liver tissues gross faster, larger and with more function in microgravity. She now has an experiment ready to fly on the International Space Station. See her website, The Chang Laboratory for Liver Tissue Engineering, at https://livertissueengineering.ucsf.edu
Tammy points out that having both microgravity and artificial gravity compartments on a space station provides the required microgravity laboratory and artificial gravity compartments for on board researchers health and comfort and also lab space for control experiments and standard analysis instruments that do not work in microgravity. This enables near real time evaluation rather than waiting weeks and months for samples returned to Earth with time delay and reentry environment potential impact on results that confuse and delay progress.
Air Launched all Reusable Spaceplane
The vast majority of payload mass to orbit will be launched on heavy lift reusable vertical launch, vertical landing rockets such as the Falcon Heavy, New Glen and Starship. Air launched spaceplanes can launch small and medium payloads to their operational orbit on demand with the ability to fly to the launch window in the sky and avoid weather, traffic and hostile action. The same system can perform unwarned intelligence, surveillance and reconnaissance (ISR) missions over the most critical denied airspace. Over time one hour worldwide point to point delivery will develop as a new commercial business.
Many spaceplanes have been proposed over the last 50-60 years and the X-15, Space Shuttle and X-37B became operational. These were all either suborbital or require large rocket boosters. Spaceplanes unlike air-breathing aircraft must include their oxidizer which makes up approximately 70% of their gross weight. This concept is towed to launch altitude by a missionized cargo aircraft that carries the oxidizer on board and transfers it to the spaceplane at altitude. Oxidizer transfer is the enabler for single stage all reusable spaceplanes.
OSTP Cislunar Technology Priorities Request for Information Response Summary
RFI Question:
“What research and development should the U.S. government prioritize to help advance a robust, cooperative, and sustainable ecosystem in cislunar space in the next 10 years? And over the next 50 years?”
Short answer for the next 10 years:
We believe that the U.S. government priority for cislunar space in the next 10 years is the establishment and distribution of electrical and thermal power at the giga-Watt (GW) level. Multiple power stations should be strategically established in cislunar space. They can be large solar energy farms and or nuclear fission and later fusion energy power plants. The energy can then be beamed by laser or microwave to the point of use. This will enable a cislunar space economic domain with cislunar resource utilization, cislunar manufacturing, thermal rocket transportation systems as well as expanded scientific research and exploration. The U.S. government should also sponsor the development and demonstration of beaming cislunar power to Earth.
Short answer for the next 50 years:
Power needs will continue to grow exponentially over time. The U.S. government should add power stations to meet the growing demand. During this period the U.S. government should also facilitate moving dangerous or polluting industrial activities off Earth and into cislunar space by sponsoring pilot plants to reduce risks sufficiently for private investment. During this period the U.S. government should lead the establishment of a cislunar space port for the assembly, outfitting, departure, return and refurbishment of crewed and uncrewed exploration systems for Mars and beyond. During this period the U.S. government will also need to patrol cislunar space much like our Navy patrols the oceans to protect free trade, access and the rule of law.
The full RFI response is available upon request.
OSTP Sustainability of Microgravity R&D Request for Information Response Summary
Question 1:
“What should be the United States' vision for the future of microgravity research?”
Short answer to question 1:
We believe the United States’ vision for the future of microgravity research should be to maintain the continuity of current research in low Earth orbit (LEO) while expanding research, exploration and commercial development utilizing all LEO and cislunar space resources for the betterment and expanded opportunities for all on Earth.
Detailed answer to question 1:
In order to meet this vision, future research platforms, need to be expanded to include longer duration experiments with pristine microgravity not interrupted or jolted by visiting spacecraft docking or system vibration and noise. Samples from the microgravity experiments need to be evaluated near real time and not have to wait weeks or months for down mass transportation to a terrestrial laboratory. Living arrangements need to accommodate expert researchers and technicians and must provide for their health and comfort for both short and long durations. On demand communication to experts and loved ones on Earth must be available for crew wellbeing but also to transfer work to Earth facilities. Sustainable logistics from and back to Earth and eventually from cislunar resources need to be reliable and affordable. Human-machine interfaces need to be built in to transfer work from expert researchers to automated production with mostly maintenance and upgrade technicians on board when required.
Question 2:
“What should be the long-term microgravity research goals for U.S. presence in LEO?”
Short answer to question 2:
Certainly, LEO microgravity research will be required for the foreseeable future due to the close proximity, lower transportation costs and manageable radiation hazards inside the Van Allen belts. Our long-term goals in LEO should be the preparation and support for the broader cislunar research, exploration and commercial development vision discussed previously. In addition, the U.S. should support the rule of law, traffic control, debris mitigation, communication, transportation, power and logistics for LEO commercial development
Microgravity and Artificial Gravity Human Health and Wellbeing:
A critical goal that can and should be accomplished in LEO is a clear understanding of human health and wellbeing in microgravity and partial to full Earth artificial gravity We have good data from the MIR space station and especially from the ISS on the effects of microgravity but almost no knowledge of the effects of partial gravity or the human tolerance for gravity gradients and rotation rates in spinning artificial gravity platforms. This knowledge is required for the design of future cislunar platforms, lunar bases, Mars missions, etc. The current estimate for the maximum rotation rate humans can tolerate due to the Coriolis effect and gravity gradient is 4 revolutions per minute (rpm). To achieve full Earth gravity at 4-rpm requires a spin radius of 55-meters. Of course, the 4-rpm limit is an estimate and could vary widely for a diverse human population and crews may be able to be conditioned for higher spin rates. The gravity gradient for a six-foot-tall standing person rotating at 4-rpm in a 55-meter spin radius platform is 9.81 to 9.48-m/s2 or a 3.3% change. We need to understand these effects much better and can do it in an experimental spinning space station before committing to the design of large LEO, cislunar or exploration systems. A full circular hoop configuration makes sense for the future, especially if it is made in space using lunar and perhaps near-Earth asteroid materials, but the gravity level, spin rate and spin diameter effects need to be well understood before proceeding with such a huge investment. Pristine microgravity compartments with low impact docking ports and low vibration systems are required for microgravity research and made in microgravity production. Artificial gravity habitats provide crew health and comfort as well as workstations to manage the microgravity activities, control experiments and analytical laboratory instruments that cannot function in microgravity. This allows researchers to tend their microgravity experiments and retrieve samples for immediate comparison to artificial gravity control samples using standard terrestrial laboratory instruments such as gene sequencers, mass spectrometers, etc.
Made in Microgravity Human Organ Tissues
LEO is perhaps the best location for research, development and then production of human organ tissues for eventual use in human transplants. Early experiments indicate that growing human organ tissues in microgravity enables the growth of isotropic three-dimensional (3D) tissue structure that lay flat when attempted in Earth gravity. This improves diffusion, fluid flow and growth rate. The microgravity organoid function continues to improve over time possibly due to better fluid flow through the more 3D structure. Tissues can be grown from disassociated organ tissues or human skin derived stem cells. Since the cells have the genetic instructions in their DNA, it is possible that the microgravity grown isotropic tissues will differentiate and form a vascular system and sinew sufficient for transplant. Liver, retinas and other organs have potential and could make significant impact on human health on Earth as transplant tissues or treatments. Liver tissues are particularly interesting due to the dire need and the natural ability of livers to regenerate to full size with only a one third adult liver mass transplant tissue. LEO is a great location due to the lower up and down mass transportation costs, low latency for automated system telemetry and manageable space radiation due to the Van Allen belts. With some Government stimulus, made in LEO human organ tissues could transition nicely to commercial operations.
Question 3:
“What are the top critical research, development, or operational needs required to ensure a smooth transition between the International Space Station and future commercial LEO microgravity platforms and realize the ideal future of microgravity research?”
Answer to question 3:
Time is running out to develop and deploy the next LEO research platforms before the ISS is retired. Perhaps a number of simple, optionally inhabited platforms make sense. The B-21 bomber is being designed to be optionally piloted and serves as a model for an optionally inhabited LEO research platform. A gap in LEO R&D waiting for an exquisite new platform would be terrible. Do not let perfect be the enemy of on time and good enough.
Question 4”
“What would be the most effective role of the U.S. government to ensure sustained LEO microgravity R&D following the retirement of the ISS?”
Short answer to question 4:
The U.S. government most effective roles to ensure sustained LEO microgravity R&D are:
Enforce the rule of law with international partners
Manage orbital traffic control
Establish and enforce debris mitigation treaties and methods
Manage and ensure available and open communication
Establish and enforce cyber security treaties and methods
Improve safety and reliability through expert objective accident prevention and investigations
In addition, the US government should promote commercial research and made in LEO economy via:
Promote affordable space transportation as an anchor customer
Promote electric and thermal power and other logistics as an initial provider and later as an anchor customer
Stimulate promising LEO commercial research and development through seedlings and other grants and by providing an anchor customer
Question 5:
“Should the U.S. government continue to sponsor a national lab in LEO after ISS transition? If so, what would be the best model(s) for a LEO national lab?”
Answer to question 5:
Possibly. A cislunar national lab and lunar national labs makes sense. LEO R&D can likely be transferred to commercial outfits. A possible exception is the Microgravity with artificial gravity research platform.
The full RFI response is available upon request.
Partners and Supporters
Ansys/AGI System Tool Kit (STK) simulation software and support
McKinney and Associates Optimal Control by Implicit Simulation (OTIS) for trajectory optimization
SpaceWorks QuickShot for trajectory optimization
NASA Glenn Chemical Equilibrium and Applications (CEA) software and support
SolidWorks/MLC-CAD Computer Aided Design (CAD) software and support
Saint Gobain pressure activated seals and design support
The Chang Laboratory for Liver Tissue Engineering at the University of California San Francisco
Get in Touch
We are looking for interns or retirees who would like to participate in the STK simulations, CAD designs and hardware tests and demonstrations. We do not have any employment opportunities at this time.